Animal Movement
How long have you been sitting at the computer?
Are you stuck to the chair yet?
Okay, we don't have to focus on how long you've been sedentary, but when you finally decide to move, how will you move and what will be the reason? Humans use different types of movement depending on what they're doing and the environment that they are doing it in. Humans can walk, run, jump (on one leg or two, crawl, roll, skip, along with many other motions, and each movement has its time and its place. For example, if you were being chased by a Canada goose you would probably sprint faster than if you were being chased by a snail. We each have reasons for our various types of movements, and other animals are no different; they each move in a particular way and for a particular reason.
1) Density: Density is a measure of an object's mass in comparison to its volume. Water has a much higher density than air which means that animals must push back against the water in order to propel themselves. This is why water animals often have paddle-like fins that are efficient at pushing against the dense water. This is also why a streamlined shape is beneficial as it can cut through the water like a torpedo.
2) Buoyancy: It might seem strange to think that fish need to actively work to keep from sinking, but gravity applies to them too. Buoyancy is the ability to stay afloat, and it is all a matter of density. In simple terms, things with a higher density than water will sink, things with a lower density than water will float.
As mentioned above, water has a higher density than air, which is why we can (mostly) float in a pool and why Wile E. Coyote always falls through the air…ultimately. In a deep body of water, it is very dangerous to sink too low due to changes in temperature and pressure that occur as they get deeper. An animal's up and down movement is just as important as its front-to-back and left-to-right movements.
3) Current: Some bodies of water also contain water currents that can be quite strong. These currents can either be harmful or useful depending on how the animal moves or if the animal moves.
If the tail swishes equally in each direction, the sideways forces cancel each other out and the fish will move straight forward. This is called body-caudal movement, and this is the primary type of movement for most fish. It's also the way Michael Phelps prefers to get around.
The amount of body undulation can vary amongst different types of fish. For example, an eel will utilize its entire body in creating this wave of movement since it has no isolated caudal fin. A trout, on the other hand, will have a relatively stationary head region, and relies heavily on its rear half and tail. The dorsal fin is also involved in movement by providing balance and maintaining the fish in an upright position. Only dead fish swim with their belly's up.
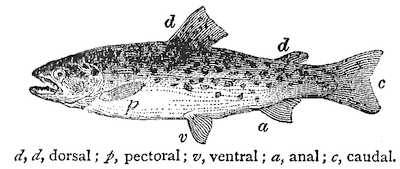
Diagram of fish fins.
Another type of movement is called median-paired fin movement, and this movement utilizes the side fins which are called pectoral fins. Movement done with the pectoral fins helps to steer the fish right or left. This type of movement is often the primary movement for tiny fish, and if you have ever been snorkeling then this quick darting directional changing movement might sound familiar. It is also one of the reasons it's so difficult to bowfish guppies. Don't try it at home.
The pectoral fins can also help maintain buoyancy in a process called dynamic lift. This is particularly important in sharks. Picture a shark; their well-developed pectoral fins stick out stiffly to the side like the wings on an airplane. In fact, they work just like an airplane's wings. By maintaining them at an appropriate angle as they move forward they can displace water downward. This generates upward lift for the shark. Sharks and some other fish use this lift to counteract gravity. Just like an airplane, if a shark stops moving forward it will stop generating lift and it will drop. This means that sharks can never stop moving forward in the water or their pectoral fins will stop providing the proper lift and they will sink to the depths like a plank-walking pirate.
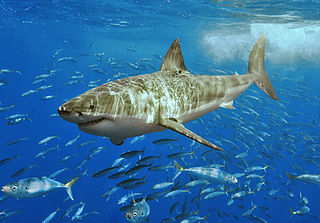
A great white shark swimming amongst other fish. Notice the pectoral fins sticking out at a fixed angle like the wings of an airplane. Image from here.
Another method used by fish to promote buoyancy is a swim bladder. The swim bladder is an organ located underneath the dorsal fin that consists of one or two air sacs. Fish can control the import and export of gases filling these sacs to control their vertical movement. More gas in the swim bladder reduces the fish's density. Since the density of the water remains the same, a fish with decreased density will become more buoyant. Sharks and rays do not have a swim bladder, which is why they have to rely on dynamic lift to keep them buoyant.
Unfortunately, the ability to stop swimming and lazily maintain buoyancy comes with a price. Fishermen have learned to exploit the special nature of the swim bladder in order to locate fish deep under the water. Fish locator equipment works by sending out sonar signals that reflect off of the air in a fish's swim bladder. The fish locators then detect the reflected signal. They can then analyze the signal and predict what type of fish it is.
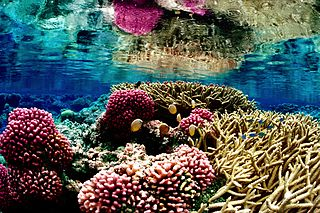
Coral reef community. Most species of coral are sessile animals which spend most of their lives anchored in one spot. Image from here.
A common feature among aquatic invertebrates is a hydrostatic skeleton. Instead of having bones they have a fluid filled cavity surrounded by muscles. The volume of fluid inside the cavity is relatively constant, and when the muscles contract in one dimension, it results in a bulge in another dimension.
Think of these critters as the water balloons of the sea. If you squeeze one side of the balloon, the opposite side will bulge. When muscles contract around the fluid-filled cavity the same thing happens and generates shape change that can lead to locomotion. A few of the animals that utilize a hydrostatic skeleton are the following: jellyfish, starfish, and sea urchins.
There are many types of jellyfish, and some of them spend all of their time floating and moving with the water currents. Others can actively contract their muscles and force water downward out of their body like a jet, thus propelling them upwards in a gentle bobbing motion. The infamous tentacles of a jellyfish are not a major player in locomotion, instead they function to deter predators and capture prey. Many humans have learned about this the hard way. Ouch!
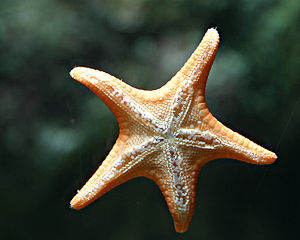
The underside of a starfish. Notice the tiny white tube-like feet.
Starfish can also move by muscle contractions that force water out of their body. However, as they are usually surface-bound, they do not move by simple jet propulsion like the jellyfish. Starfish have tiny tube feet structures on their underside. They function like suction cups that can attach and detach to the inhabited surface. In order to move, the starfish contracts its muscles to force water into its feet so that they extend. In a coordinated manner of detaching, contracting, extending, and reattaching the starfish is able to "walk" along surfaces; sometimes as quickly as 1 meter per minute!
As shown here, sea urchins move in a similar manner, the caveat being that their tube feet must extend farther than their spines in order to be useful. Sea urchins can also individually manipulate their spines for locomotion as well. Both starfish and sea urchins often need active movement if they get caught in a tidal pool.
The key to neutral buoyancy is having the same density as water. If we were to fill a giant model whale with water, it would have the same mass as a real whale. If they were both the same size, that is. That's one big water balloon. Whales have a lot of heavy parts like muscle and bones, but they reduce their overall mass by maintaining a very high fat content in their bodies, including a layer beneath their skin called blubber.
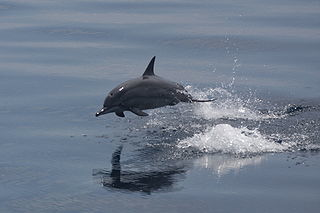
A dolphin jumping. Dolphins are well known for their playful movements. They particularly enjoy jumping and dancing alongside the bow of a ship. Image from here.
Dolphins and whales move primarily from the thrust of their caudal fin. This is unlike fish since the caudal fin is oriented in a perpendicular direction to the fish tail fin. The tail contains two lobes known as flukes which produce strong upward and downward strokes and can propel dolphins through the water at speeds near 30 mph. Just as in fish, the dorsal fin (some whales don't have one) provides balance, and the pectoral fins aid in steering. Both animals have also been known to leap above the water in an act called breaching. Dolphins are particularly well known for their playful leaping and acrobatic abilities, and are often seen bow-riding.
A different type of marine mammal is the sea otter, which is one of the few marine mammals that do not utilize high fat content to promote buoyancy. Instead, it relies on the large volume of air in its lungs, which are nearly 2.5 times larger than a similarly sized land-mammal's lungs.
The sea otter also has appendages that are more like legs with feet than they are like fins. This is because the sea otter can spend time on land when it needs to, although it does spend nearly all of its time in the water. Its feet are webbed to make them more efficient at paddling, and together with its broad tail, it uses a vertical thrusting motion to propel itself. The front feet are usually tucked closely in to make it as torpedo-like as possible. A favorite activity of the sea otter is to float on its back, which it likely does to conserve energy.
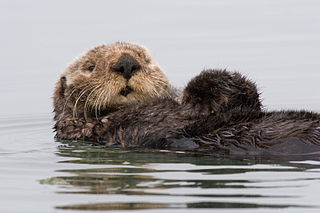
A sea otter floating on its back. Floating on their backs helps sea otters to conserve energy. Image from here.
In order to understand flight, we can start with a brief explanation of how airplanes fly, in basic terms. As we mentioned for sharks, the angle of the wings is a very important part of what makes a plane fly. The shape of the wings, called the airfoil, causes gases moving around the animal to move in a certain way which causes more force pushing up from underneath the wing than is pulling down on it. This upward force perpendicular to the wing is called lift. Still confused? Don't worry about it. It takes some serious knowledge of physics to understand exactly how the gases are moving around an airfoil. In fact, according to NASA, lots of people who think they understand flight, but they really don't.
For simplicity, just know that a force is pushing underneath the wings and lifting it up. Stick your arms out to your sides with your palms facing the floor. Now, imagine a friend taking his or her index fingers and poking you in the middle of your palms. The force that pushes directly into your palms is going to be perpendicular to the air flowing over your hands, and that will be the lift force. Lift is required for everything that flies, and in order for an animal to fly it must generate enough lift to counteract gravity's pull.
Different airfoils cut through the air differently, and in the picture below you can see the different types of airfoils used by different types of airplanes and animals. The speed of the animal is a crucial determinant of lift, and if the air stops moving over the wing then lift cannot be produced. The type of airfoil that an animal has determines how fast it needs to move in order to generate lift.
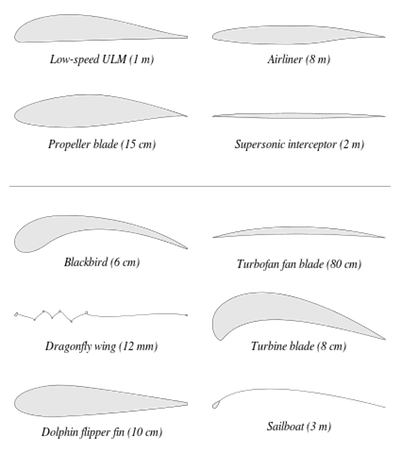
Diagram of different types of airfoils used by animals and man-made things
Gliding can either be a gradual descent or a steep descent, depending on the angle of the bird's wings. Sometimes birds can glide from a high place and use gravity to increase their forward motion. Other times they can gather speed by flapping and then switch to gliding, kind of like pedaling a skateboard and then riding on it. A gliding bird can also take advantage of wind and thermal air currents to maintain their lift, and this is called soaring. Soaring is the best movement for a bird because it uses the least amount of energy.
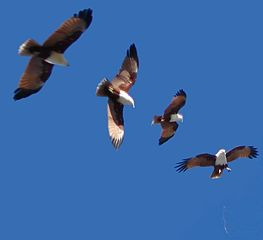
A flying bird. This birds is not flapping its wings, but it either gliding or soaring. Image from here.
Flapping uses much more energy than soaring, and it also involves more complex physics. When a bird pushes down with its wings it is actually pushing slightly down and slightly backwards, so that the force it generates is lifting it upwards and forwards. Sounds simple enough, but a bird doesn't just pump its wings up and down, it is also twisting and folding them. The twisting motion directs the angle of attack of the airfoil to alter the lift and drag so that the bird can change speed or direction. The folding motion is used on the upward flap to make the wing shorter, but why would that help?
During the downward flap the bird is lifting itself upward and forwards, so what is happening on the upward flap? It does just the opposite and forces the bird downwards and backwards. Anytime the bird is flapping up it creates the wrong type of force. Shorter wings produce less force. By making the wing shorter on the up flap, it ensures that the down/backward forces will always be less than the up/forward forces. The net result is the bird moving upwards and forwards.
In order for a bird's wings to function, its feathers are of the utmost importance. The arrangement of feathers comprises the airfoil, and birds have many types of specialized feathers distributed across their wings.
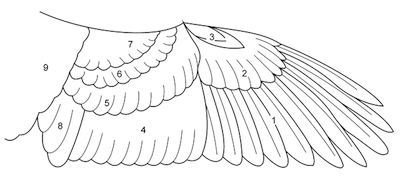
Diagram of the different types of specialized feathers a bird needs for flight 1. Primary remiges 2. Greater primary coverts 3. Alula 4. Secondary remiges 5. Greater secondary coverts 6. Median secondary coverts 7. Lesser secondary coverts 8.Tertiary remiges 9. Scapulares.
However, the feathers are not the only part of a bird that make it capable of flying, and birds have several adaptations which make them as light as possible. First of all, most birds also have hollow bones, which are important because a bird's skeleton is very strong and dense, and hollow bones decrease the weight. Birds also do not have to carry around heavy babies, as they are oviparous and their cute little bird babies develop in laid eggs. Birds also eat food that is high in calories and can be digested quickly. This allows them to eat, process, and excrete waste rapidly without carrying it around. The heaviest known flying bird is the kori bustard. This birdie can weigh up to 44 lbs. There are heavier birds, like the ostrich, emperor penguins, and Big Bird; however, they are flightless.
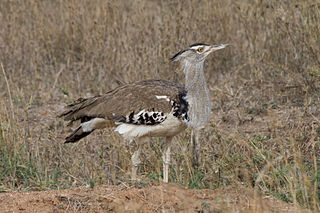
The heaviest flying bird, the Kori Bustard can weigh up to 44 lbs. Image from here.
Most insects have two pairs of wings that flap together. It has been difficult to study insect flight due to the small size and extremely fast rate of insect wing beats. The tiny gnat-like midge, like the ones shown below, can beat its wings 1,046 times per second. Can you even imagine something moving that fast?
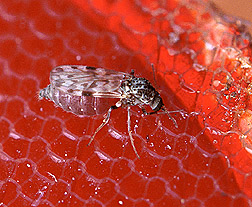
A type of midge. Some types of midge can beat their wings over 1,000 times per second.
In the last decade, high speed digital photography has made it possible to analyze the wing movement of individual insects. The honeybee, for example was long thought to fly against the laws of physics, but a study in 2006 finally uncovered the dynamics of bee flight. As more and more insects are filmed we will learn the details about the aerodynamics of insect flight.
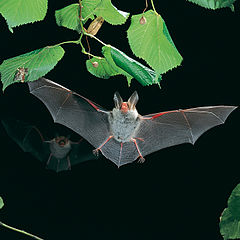
A flying bat. Notice how the bones in the wing tips almost look like hands with long thin fingers spread wide open.
Bats have long thin wings than are covered in skin. They are able to generate lift via their airfoil similarly to birds, but a bat's airfoil is more curved than a bird's, which gives it more lift power in straight forward flight. The underlying bone structure is shaped more like a splayed out human hand than it is shaped like a bird's wing. It has over two dozen joints in it that allow them to twist and fold their wings in unique ways. This specialized wing control allows them to accomplish a plethora of unique and dynamic flight patterns. For example, bats can change direction 180 degrees very quickly, which is a rare capability among fliers.
The other flying mammals are all gliders, which means they are incapable of flapping and rely on gravity to aid in their flight. There are 65 identified species of flying mammals, most of which are different species of flying squirrel. These creatures are nocturnal and have specialized gliding membranes called patagia. Each thin patagium is two layers of skin with connective tissue, muscles, and nerves in between. The skin covering on a bats wing is actually a form of a patagium.
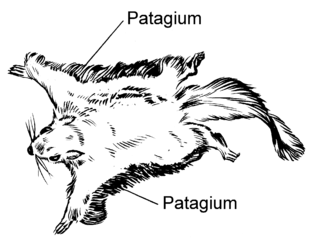
By spreading their limbs at right angles to their body, they create a rough airfoil. This allows them to gracefully glide downward using gravity while generating some lift from their "wings." They even have a braking system so they can slow down for landing. By pointing their limbs forward towards their destination, the patagia create a parachute type structure which fills with a burst of air like a parachute that has just opened and they slow down. The aerodynamics of this maneuver also gives them a final bit of lift, and they can safely land and grab on with their claws. Japanese giant flying squirrels can glide over 100 meters which is the length of a football field. Check out these daredevils stealing some ideas from our patagia-bearing friends.
In fact, without a piece of sturdy ground to push against, most animals would have a hard time moving. Picture yourself in a bouncy castle. We know it's hard to do. If you need to visit the local bouncy barn, we'll wait. You'll find that the squishy ground does not provide a sturdy surface to push against and walking is difficult.
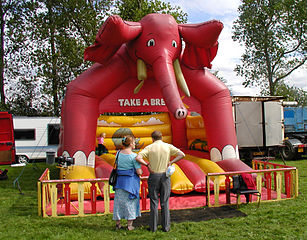
A bouncy elephant. Walking on a squishy surface is difficult since there is not a stable surface to push off from.
The method of walking, bearing, or carriage that an animal has is called its gait. One way to separate gaits it based on how many limbs the animal has. Limbs are important structures in animal movement, and they can determine what type of movement an animal is capable of.
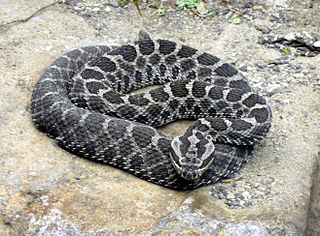
A close up view of a rattlesnake. Notice the scales covering the snake. A snake needs scales to help it slither.
Snakes have scales that aid them in moving forward, although it is difficult for a snake to move directly sideways. A snake gradually redistributes its body weight and uses its scales to provide thrust off of different points on the ground. There are four primary ways that snakes move: concertina, serpentine or lateral undulation, sidewinding, and rectilinear. That's a lot of movements for an animal without limbs.
Snails lack the scales that snakes have, and instead they expel mucus on which they slither. Similar to snakes, they move by utilizing a wave of muscular movement, and their adhesive mucus provides the friction necessary to avoid backsliding. Scientists have been studying the mucus-driven movement of snails to better design endoscopes, which must be inserted into the mucus-lined respiratory or digestive tracts.
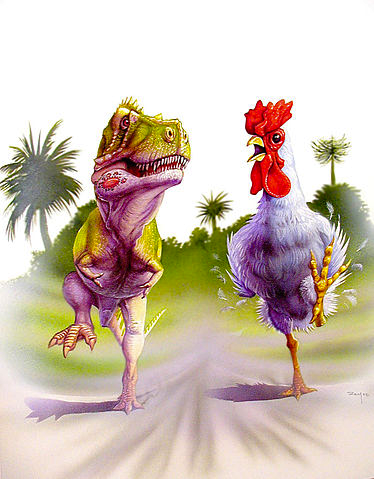
Cartoon illustrating the similarities between Tyrannosaurus rex and birds. Both are bipeds. Image from here.
Bipedal walking requires balance and the interplay of one foot pushing into the ground while the other foot propels forward. Some animals can also run, which is similar to walking, but in running there are moments when neither foot is touching the ground.
All birds are bipedal. For flightless birds like the ostrich and the emu this is their only way to move. Walking requires strong thigh musculature, and although birds may have a developed a negative reputation for having spindly "bird legs," they actually have large thigh muscles hidden close beneath their bodies. These strong muscles enable them to walk and hop around. Just check out the muscle on those turkey legs. If they weren't dinner, they'd probably be at the gym.
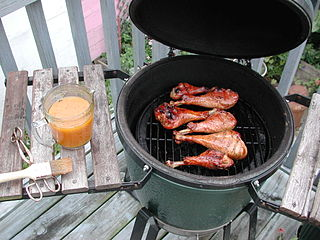
Turkey legs on a grill. Notice the thick upper thigh muscle. Image from here.
Humans walk differently than birds do because our bodies are not aligned the same. We stand fully upright with our spines vertically aligned and settled into our hipbones. Birds, on the other hand, have a spine that sits on their hips more like a T-shape with the neck and tail sticking out on either side of the central hip joint like the spine is planking on the hips. Remember planking? Weird.
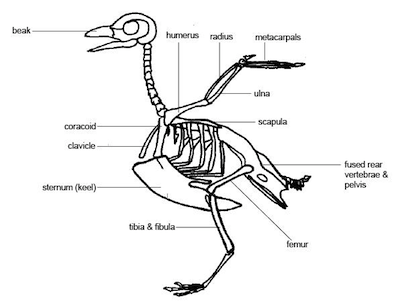
Diagram of a bird skeleton. Notice how the spine and rib cage are nearly perpendicular to the hips and legs. Humans are also bipeds, but they have a spine and rib cage that are in a straight line with their legs. Image from here.
Kangaroos are non-primate mammals that have adopted bipedalism, although they have not developed their forelimbs as much as birds and humans have. They also do not share the traditional walking gait of humans and birds and instead rely on hopping to get around at faster speeds. While grazing, a kangaroo's walking gait is neither bipedal nor quadrupedal. Its motion is pentapedal, where its tail acts as a fifth limb. It is able to form a tripod with its forelimb and its tail while its hindlimbs hop forward.
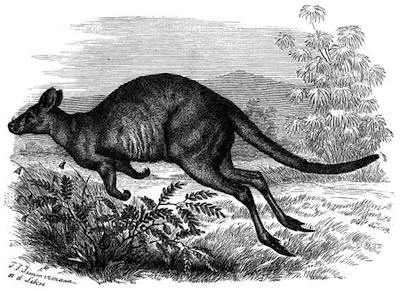
A kangaroo hopping on two large hind legs and using a tail for balance.
1) Trot: Diagonally paired legs (such as front right and rear left) touch the ground simultaneously.
2) Bounding: Front (or back) legs touch the ground simultaneously.
3) Pace: Left (or right) side legs touch the ground simultaneously.
4) Walk: Each foot hits the ground in rotating order, front left, back right, front right, and back left, with three feet on the ground at all times.
5) Rotary gallop: Front feet hit at almost exactly the same time and then the back feet hit at almost exactly the same time. If the front feet hit right then left, then the back feet hit left then right.
6) Transverse gallop: Front feet hit at almost exactly the same time and then the back feet hit at almost exactly the same time. If the front feet hit right then left, then the back feet hit right then left.
7) ) Canter: Three beat type of movement. One foot hits, then the two remaining feet hit which are diagonal from each other, and then the final foot. For example—front right, then front left and back right, then back left. 8) ) Pronk: all four legs hit simultaneously. Yes, it's as cool as it sounds.
Since some animals use certain gaits but not others, animal trackers can use that information when they look at footprints. By looking at the shape, size, and the pattern of tracks, we can determine what kind of animal left them, how big it is, and how fast it was moving when it passed though. Hint: a flying animal will leave no tracks.
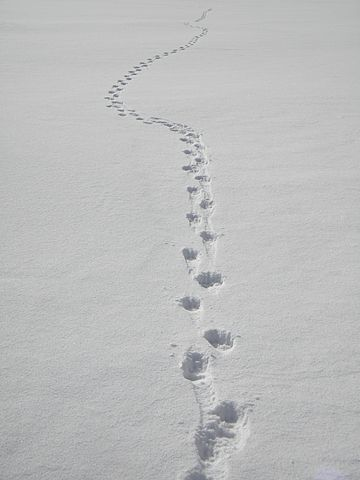
Tracks in the snow. Tracks can be examined to determine the gait that an animal had. Image from here.
Scientists wondered what the benefit was for spiders to have eight legs instead of the traditional six legs. In the end, it took a dark room and a camera to figure it out. Amazingly, spiders are capable of walking flawlessly on six legs…in the dark. Almost like a blind person using a cane, they use their front pair of legs as antennae when they move in the dark.
There is a hindrance of having as many legs as a centipede or a millipede; it takes more coordination to move them all. Millipedes are relatively slow, but more legs means more power for pushing off the ground and for burrowing. Although the names centipede and millipede imply 100 legs and 1000 legs respectively, centipedes and millipedes don't actually have that many legs. Centipedes actually have between 30-362 legs and millipedes have between 36-400 legs, depending on the species.
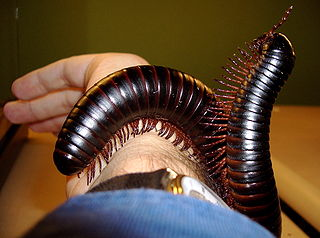
A Giant African Millipede. Look at all those legs. Image from here.
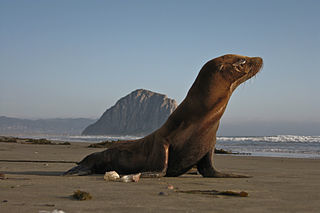
A sea lion pup on the beach. Sea lions can move on land or in the water, but they are built for water movement. On land they have to move very slowly. Image from here.
At the other end of the food chain, tiny fish have developed defensive movements. Median-paired fin movement allows fish to dart quickly in different directions. This helps them to avoid predators which cannot change direction as quickly.
Schooling or shoaling is another way that tiny fish can move to evade predators. Both tactics allow them to confuse predators with poor eyesight, and they can also provide safety in numbers since many eyes on the lookout makes it easier to identify potential predators.
Over time, predators and their prey can adapt counter-tactics to each other. One such tactic is demonstrated in an event known as the sardine run. Every summer, a large population of sardines can be found off the coast of Africa. Predators know that the sardines arrive every year, so they travel to the site, too. The sardines cluster together to protect themselves, but the predators have learned to benefit from this shoaling. They work together to round-up groups of sardines into tight clusters called baitballs. Then, the predators patrol the perimeter and take turns rushing in to satiate themselves at the swimming buffet. We bet there are some foods you wish would appear in large numbers every summer.
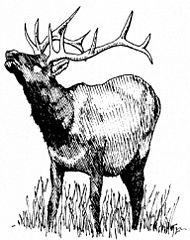
A male elk. Elk crash their antlers together in a physical competition for mating rights.
In many species, males compete with each other physically. For example, elk bound towards one another and crash their antlers together as they fight for mates. The bounding movement allows the elk to be more stable as he collides with his challenger, because it means he will dig in both front or back feet simultaneously, and ensuring greater balance.
Rather than fighting, many species of birds have complex mating rituals involving bizarre movements intended to draw attention of available females. Talk about lovebirds. Birds often fluff and displaying their vibrant plumage, although sometimes it can involve intricate dance-like movements as well. These guys have the moves like Jagger.
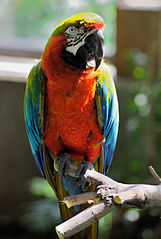
A colorful bird. Male birds are often brightly colored and perform complex movements to attract females. Image from here.
Many birds seek a warmer climate during the cold months, and, of them, bar-tailed godwits have the longest non-stop flight of any bird. By gliding on air currents and utilization of flapping flight, they have been known to fly from Alaska to New Zealand, a distance of 11,690 kilometers (or 7,263 miles) to take advantage of fair temperatures. In many parts of the United States and Canada, the crisp autumn air is filled with migratory Canada Geese in their classic V-formation. The flying V-formation is cost effective for birds traveling long distances because all birds can enjoy some of the lift caused by the lead bird. Flocks will rotate the front flier so that the burden of flying in the tiresome lead spot is shared. The flying-V is also one of the best offensive hockey maneuvers. Just ask the Mighty Ducks.
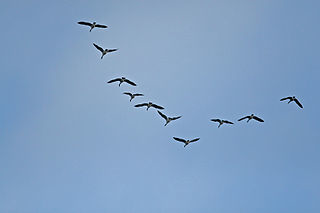
Canada Geese flying in a classic V-formation. Birds fly in this formation to conserve energy as a group. Image from here.
Caribou migrate similarly to birds except that they temporarily seek a cooler climate during the hot months rather than temporarily seeking a warmer climate during the cold months. They walk 965 km (600 miles) north during the summer months to feed on grasses of the tundra while avoiding the bloodsucking insects of warmer climates. Not a bad plan if you hate mosquitos.
Salmon have an even more complex migration pattern, as they travel to a special location for spawning. Utilizing strong body-caudal movement they propel themselves upstream against the current to spawn. Magically, they return to the exact stream where they were hatched. The distance and current alone make this a daunting task, but the truly amazing feat is when they propel themselves up a waterfall. Unfortunately, if there is a bear waiting at the top their effort will be wasted.
2) While you're still thinking about flying sharks, could a shark moving super fast fly like an airplane? The short answer is, probably not. The shark's fins are angled in a way that generates lift when traveling through dense salt water, but the forces generated when moving through air would not be sufficient. Sharks can propel themselves up and out of the water momentarily, but fear not—flying sharks will remain in the Hollywood prop closet and out of the friendly skies. Or will they....
Are you stuck to the chair yet?
Okay, we don't have to focus on how long you've been sedentary, but when you finally decide to move, how will you move and what will be the reason? Humans use different types of movement depending on what they're doing and the environment that they are doing it in. Humans can walk, run, jump (on one leg or two, crawl, roll, skip, along with many other motions, and each movement has its time and its place. For example, if you were being chased by a Canada goose you would probably sprint faster than if you were being chased by a snail. We each have reasons for our various types of movements, and other animals are no different; they each move in a particular way and for a particular reason.
Water-Based Movement
There are certain things that animals living in water need to take into consideration that land dwellers do not. Keep these in mind as we discuss specific animal movement.1) Density: Density is a measure of an object's mass in comparison to its volume. Water has a much higher density than air which means that animals must push back against the water in order to propel themselves. This is why water animals often have paddle-like fins that are efficient at pushing against the dense water. This is also why a streamlined shape is beneficial as it can cut through the water like a torpedo.
2) Buoyancy: It might seem strange to think that fish need to actively work to keep from sinking, but gravity applies to them too. Buoyancy is the ability to stay afloat, and it is all a matter of density. In simple terms, things with a higher density than water will sink, things with a lower density than water will float.
As mentioned above, water has a higher density than air, which is why we can (mostly) float in a pool and why Wile E. Coyote always falls through the air…ultimately. In a deep body of water, it is very dangerous to sink too low due to changes in temperature and pressure that occur as they get deeper. An animal's up and down movement is just as important as its front-to-back and left-to-right movements.
3) Current: Some bodies of water also contain water currents that can be quite strong. These currents can either be harmful or useful depending on how the animal moves or if the animal moves.
Fish
Fish, the primary water dwellers, have a skeleton and muscles that help them move their fins, just like our muscles and bones help us to get down with our bad selves. The fish tail is called a caudal fin, and it works together with rows of muscles along the length of the fish's body which contract in a cascading movement. Imagine Jerry Lee Lewis running his fingers across a piano hitting each key in succession, "Great Balls of Fire" style. This is how the muscle contraction spreads along the length of the fish on one side followed by the other causing the tail to swish from side to side.If the tail swishes equally in each direction, the sideways forces cancel each other out and the fish will move straight forward. This is called body-caudal movement, and this is the primary type of movement for most fish. It's also the way Michael Phelps prefers to get around.
The amount of body undulation can vary amongst different types of fish. For example, an eel will utilize its entire body in creating this wave of movement since it has no isolated caudal fin. A trout, on the other hand, will have a relatively stationary head region, and relies heavily on its rear half and tail. The dorsal fin is also involved in movement by providing balance and maintaining the fish in an upright position. Only dead fish swim with their belly's up.
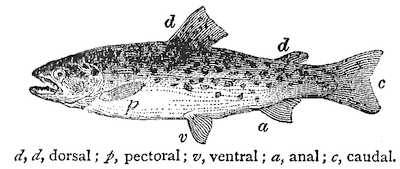
Diagram of fish fins.
Another type of movement is called median-paired fin movement, and this movement utilizes the side fins which are called pectoral fins. Movement done with the pectoral fins helps to steer the fish right or left. This type of movement is often the primary movement for tiny fish, and if you have ever been snorkeling then this quick darting directional changing movement might sound familiar. It is also one of the reasons it's so difficult to bowfish guppies. Don't try it at home.
The pectoral fins can also help maintain buoyancy in a process called dynamic lift. This is particularly important in sharks. Picture a shark; their well-developed pectoral fins stick out stiffly to the side like the wings on an airplane. In fact, they work just like an airplane's wings. By maintaining them at an appropriate angle as they move forward they can displace water downward. This generates upward lift for the shark. Sharks and some other fish use this lift to counteract gravity. Just like an airplane, if a shark stops moving forward it will stop generating lift and it will drop. This means that sharks can never stop moving forward in the water or their pectoral fins will stop providing the proper lift and they will sink to the depths like a plank-walking pirate.
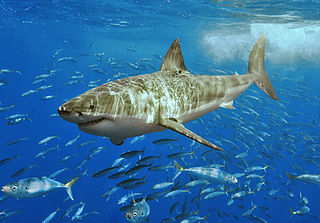
A great white shark swimming amongst other fish. Notice the pectoral fins sticking out at a fixed angle like the wings of an airplane. Image from here.
Another method used by fish to promote buoyancy is a swim bladder. The swim bladder is an organ located underneath the dorsal fin that consists of one or two air sacs. Fish can control the import and export of gases filling these sacs to control their vertical movement. More gas in the swim bladder reduces the fish's density. Since the density of the water remains the same, a fish with decreased density will become more buoyant. Sharks and rays do not have a swim bladder, which is why they have to rely on dynamic lift to keep them buoyant.
Unfortunately, the ability to stop swimming and lazily maintain buoyancy comes with a price. Fishermen have learned to exploit the special nature of the swim bladder in order to locate fish deep under the water. Fish locator equipment works by sending out sonar signals that reflect off of the air in a fish's swim bladder. The fish locators then detect the reflected signal. They can then analyze the signal and predict what type of fish it is.
Aquatic Invertebrates
Apart from fish, the water is full of other animals. With the exception of insects, most of the planet's invertebrate animals are water-dwelling, and they can move in interesting ways. That is, unless they are one of the invertebrates such as coral and sponges that exhibit sessility. These animals have very little movement over the course of their lives. They are so immobile that they would probably not even notice if someone cast the "Petrificus Totalus" spell. Often the only movement they have is floating with the current during their infancy. Once they adhere somewhere they stay there forever, allowing the currents to bring food to them.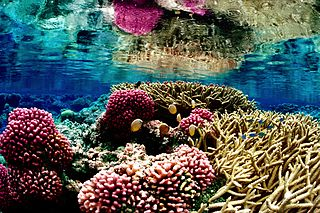
Coral reef community. Most species of coral are sessile animals which spend most of their lives anchored in one spot. Image from here.
A common feature among aquatic invertebrates is a hydrostatic skeleton. Instead of having bones they have a fluid filled cavity surrounded by muscles. The volume of fluid inside the cavity is relatively constant, and when the muscles contract in one dimension, it results in a bulge in another dimension.
Think of these critters as the water balloons of the sea. If you squeeze one side of the balloon, the opposite side will bulge. When muscles contract around the fluid-filled cavity the same thing happens and generates shape change that can lead to locomotion. A few of the animals that utilize a hydrostatic skeleton are the following: jellyfish, starfish, and sea urchins.
There are many types of jellyfish, and some of them spend all of their time floating and moving with the water currents. Others can actively contract their muscles and force water downward out of their body like a jet, thus propelling them upwards in a gentle bobbing motion. The infamous tentacles of a jellyfish are not a major player in locomotion, instead they function to deter predators and capture prey. Many humans have learned about this the hard way. Ouch!
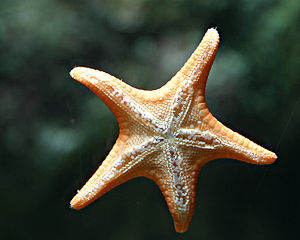
The underside of a starfish. Notice the tiny white tube-like feet.
Starfish can also move by muscle contractions that force water out of their body. However, as they are usually surface-bound, they do not move by simple jet propulsion like the jellyfish. Starfish have tiny tube feet structures on their underside. They function like suction cups that can attach and detach to the inhabited surface. In order to move, the starfish contracts its muscles to force water into its feet so that they extend. In a coordinated manner of detaching, contracting, extending, and reattaching the starfish is able to "walk" along surfaces; sometimes as quickly as 1 meter per minute!
As shown here, sea urchins move in a similar manner, the caveat being that their tube feet must extend farther than their spines in order to be useful. Sea urchins can also individually manipulate their spines for locomotion as well. Both starfish and sea urchins often need active movement if they get caught in a tidal pool.
Water Mammals
Marine mammals are larger than aquatic invertebrates. Built to survive in water, most marine mammals have a torpedo shaped build and fin-like appendages perfect for cutting through dense water like an iron chef cuts through a catfish. A key difference between them and fish is that most marine mammals are champions of neutral buoyancy. This is awesome because it means that they will neither sink nor float in the water column.The key to neutral buoyancy is having the same density as water. If we were to fill a giant model whale with water, it would have the same mass as a real whale. If they were both the same size, that is. That's one big water balloon. Whales have a lot of heavy parts like muscle and bones, but they reduce their overall mass by maintaining a very high fat content in their bodies, including a layer beneath their skin called blubber.
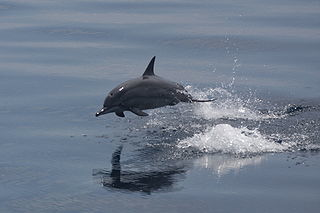
A dolphin jumping. Dolphins are well known for their playful movements. They particularly enjoy jumping and dancing alongside the bow of a ship. Image from here.
Dolphins and whales move primarily from the thrust of their caudal fin. This is unlike fish since the caudal fin is oriented in a perpendicular direction to the fish tail fin. The tail contains two lobes known as flukes which produce strong upward and downward strokes and can propel dolphins through the water at speeds near 30 mph. Just as in fish, the dorsal fin (some whales don't have one) provides balance, and the pectoral fins aid in steering. Both animals have also been known to leap above the water in an act called breaching. Dolphins are particularly well known for their playful leaping and acrobatic abilities, and are often seen bow-riding.
A different type of marine mammal is the sea otter, which is one of the few marine mammals that do not utilize high fat content to promote buoyancy. Instead, it relies on the large volume of air in its lungs, which are nearly 2.5 times larger than a similarly sized land-mammal's lungs.
The sea otter also has appendages that are more like legs with feet than they are like fins. This is because the sea otter can spend time on land when it needs to, although it does spend nearly all of its time in the water. Its feet are webbed to make them more efficient at paddling, and together with its broad tail, it uses a vertical thrusting motion to propel itself. The front feet are usually tucked closely in to make it as torpedo-like as possible. A favorite activity of the sea otter is to float on its back, which it likely does to conserve energy.
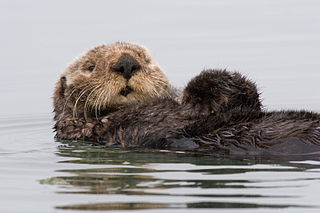
A sea otter floating on its back. Floating on their backs helps sea otters to conserve energy. Image from here.
Air-Based Movement
Humans have always been fascinated by the idea of flight. While we can leave land behind occasionally for a quick swim, flying is not something that comes naturally to us. Even Michael "Air" Jordan couldn't stay in the air forever, but it was not his fault. The human body is just not built for flight. Animals that can fly have special characteristics that make flight possible, and without these traits they would fly about as well as an elephant—Dumbo, excepted.In order to understand flight, we can start with a brief explanation of how airplanes fly, in basic terms. As we mentioned for sharks, the angle of the wings is a very important part of what makes a plane fly. The shape of the wings, called the airfoil, causes gases moving around the animal to move in a certain way which causes more force pushing up from underneath the wing than is pulling down on it. This upward force perpendicular to the wing is called lift. Still confused? Don't worry about it. It takes some serious knowledge of physics to understand exactly how the gases are moving around an airfoil. In fact, according to NASA, lots of people who think they understand flight, but they really don't.
For simplicity, just know that a force is pushing underneath the wings and lifting it up. Stick your arms out to your sides with your palms facing the floor. Now, imagine a friend taking his or her index fingers and poking you in the middle of your palms. The force that pushes directly into your palms is going to be perpendicular to the air flowing over your hands, and that will be the lift force. Lift is required for everything that flies, and in order for an animal to fly it must generate enough lift to counteract gravity's pull.
Different airfoils cut through the air differently, and in the picture below you can see the different types of airfoils used by different types of airplanes and animals. The speed of the animal is a crucial determinant of lift, and if the air stops moving over the wing then lift cannot be produced. The type of airfoil that an animal has determines how fast it needs to move in order to generate lift.
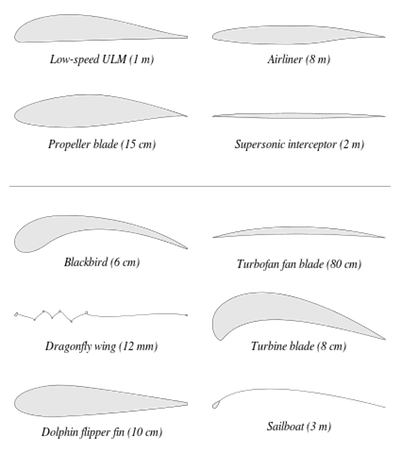
Diagram of different types of airfoils used by animals and man-made things
Birds
Birds can fly in two different ways: gliding and flapping. If a bird is gliding, it will have its wings in an extended position just like an airplane. The lift it achieves will be dependent upon its forward motion and its airfoil. For this reason, gliding birds always angle their wings downward. By doing this, the airfoil will create both lift and forward motion. Remember, the lift motion is always going to point perpendicular to the wing. Put your arms out again and rotate your arms from the shoulder so that your thumbs are slightly pointing towards the floor. Now when your friend pokes you in the palm the force will be pointing slightly forward and slightly upward.Gliding can either be a gradual descent or a steep descent, depending on the angle of the bird's wings. Sometimes birds can glide from a high place and use gravity to increase their forward motion. Other times they can gather speed by flapping and then switch to gliding, kind of like pedaling a skateboard and then riding on it. A gliding bird can also take advantage of wind and thermal air currents to maintain their lift, and this is called soaring. Soaring is the best movement for a bird because it uses the least amount of energy.
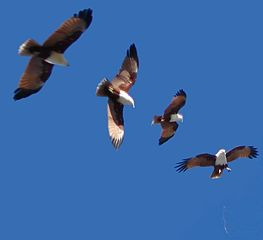
A flying bird. This birds is not flapping its wings, but it either gliding or soaring. Image from here.
Flapping uses much more energy than soaring, and it also involves more complex physics. When a bird pushes down with its wings it is actually pushing slightly down and slightly backwards, so that the force it generates is lifting it upwards and forwards. Sounds simple enough, but a bird doesn't just pump its wings up and down, it is also twisting and folding them. The twisting motion directs the angle of attack of the airfoil to alter the lift and drag so that the bird can change speed or direction. The folding motion is used on the upward flap to make the wing shorter, but why would that help?
During the downward flap the bird is lifting itself upward and forwards, so what is happening on the upward flap? It does just the opposite and forces the bird downwards and backwards. Anytime the bird is flapping up it creates the wrong type of force. Shorter wings produce less force. By making the wing shorter on the up flap, it ensures that the down/backward forces will always be less than the up/forward forces. The net result is the bird moving upwards and forwards.
In order for a bird's wings to function, its feathers are of the utmost importance. The arrangement of feathers comprises the airfoil, and birds have many types of specialized feathers distributed across their wings.
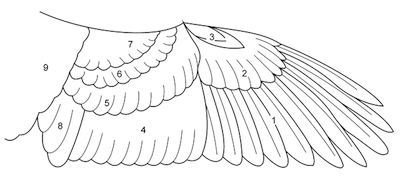
Diagram of the different types of specialized feathers a bird needs for flight 1. Primary remiges 2. Greater primary coverts 3. Alula 4. Secondary remiges 5. Greater secondary coverts 6. Median secondary coverts 7. Lesser secondary coverts 8.Tertiary remiges 9. Scapulares.
However, the feathers are not the only part of a bird that make it capable of flying, and birds have several adaptations which make them as light as possible. First of all, most birds also have hollow bones, which are important because a bird's skeleton is very strong and dense, and hollow bones decrease the weight. Birds also do not have to carry around heavy babies, as they are oviparous and their cute little bird babies develop in laid eggs. Birds also eat food that is high in calories and can be digested quickly. This allows them to eat, process, and excrete waste rapidly without carrying it around. The heaviest known flying bird is the kori bustard. This birdie can weigh up to 44 lbs. There are heavier birds, like the ostrich, emperor penguins, and Big Bird; however, they are flightless.
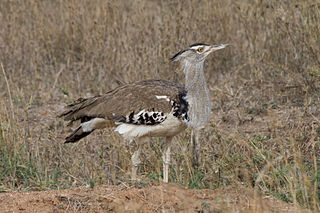
The heaviest flying bird, the Kori Bustard can weigh up to 44 lbs. Image from here.
Insect Flight
Insects are a very diverse group of animals, with more than a million described species. That means that every resident in Dallas, Texas could have a different bug infestation, with almost no overlap. That's a lot of species. Understandably, insects exhibit various types of flight.Most insects have two pairs of wings that flap together. It has been difficult to study insect flight due to the small size and extremely fast rate of insect wing beats. The tiny gnat-like midge, like the ones shown below, can beat its wings 1,046 times per second. Can you even imagine something moving that fast?
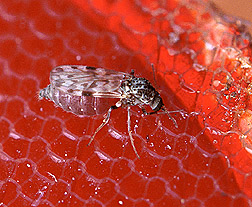
A type of midge. Some types of midge can beat their wings over 1,000 times per second.
In the last decade, high speed digital photography has made it possible to analyze the wing movement of individual insects. The honeybee, for example was long thought to fly against the laws of physics, but a study in 2006 finally uncovered the dynamics of bee flight. As more and more insects are filmed we will learn the details about the aerodynamics of insect flight.
Flying Mammals
There are several types of flying mammals, although bats are the only ones capable of true flight under their own power. To take it a step further, bats actually depend on flight more than birds and insects. Birds and insects are also great at walking or hopping, but bats are very poor at terrestrial motion and almost exclusively fly.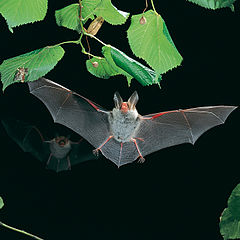
A flying bat. Notice how the bones in the wing tips almost look like hands with long thin fingers spread wide open.
Bats have long thin wings than are covered in skin. They are able to generate lift via their airfoil similarly to birds, but a bat's airfoil is more curved than a bird's, which gives it more lift power in straight forward flight. The underlying bone structure is shaped more like a splayed out human hand than it is shaped like a bird's wing. It has over two dozen joints in it that allow them to twist and fold their wings in unique ways. This specialized wing control allows them to accomplish a plethora of unique and dynamic flight patterns. For example, bats can change direction 180 degrees very quickly, which is a rare capability among fliers.
The other flying mammals are all gliders, which means they are incapable of flapping and rely on gravity to aid in their flight. There are 65 identified species of flying mammals, most of which are different species of flying squirrel. These creatures are nocturnal and have specialized gliding membranes called patagia. Each thin patagium is two layers of skin with connective tissue, muscles, and nerves in between. The skin covering on a bats wing is actually a form of a patagium.
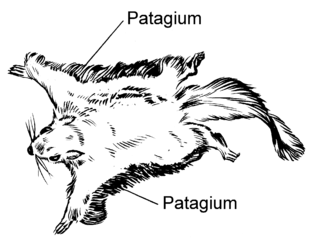
By spreading their limbs at right angles to their body, they create a rough airfoil. This allows them to gracefully glide downward using gravity while generating some lift from their "wings." They even have a braking system so they can slow down for landing. By pointing their limbs forward towards their destination, the patagia create a parachute type structure which fills with a burst of air like a parachute that has just opened and they slow down. The aerodynamics of this maneuver also gives them a final bit of lift, and they can safely land and grab on with their claws. Japanese giant flying squirrels can glide over 100 meters which is the length of a football field. Check out these daredevils stealing some ideas from our patagia-bearing friends.
Terrestrial Locomotion
Movement on land is called terrestrial locomotion. Unlike movement in water or movement in air, terrestrial movement does not have much up and down movement since the animal must always be on the ground (or on another solid object like a tree branch). Gravity roots terrestrial animals to the ground, and they must harness frictional forces between their bodies and the sturdy ground in order to move.In fact, without a piece of sturdy ground to push against, most animals would have a hard time moving. Picture yourself in a bouncy castle. We know it's hard to do. If you need to visit the local bouncy barn, we'll wait. You'll find that the squishy ground does not provide a sturdy surface to push against and walking is difficult.
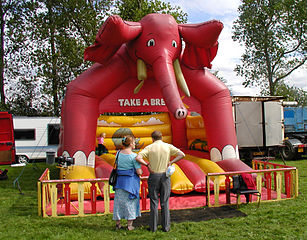
A bouncy elephant. Walking on a squishy surface is difficult since there is not a stable surface to push off from.
The method of walking, bearing, or carriage that an animal has is called its gait. One way to separate gaits it based on how many limbs the animal has. Limbs are important structures in animal movement, and they can determine what type of movement an animal is capable of.
Limbless
Slithering is the most common gait for limbless animals such as worms, snails, and snakes (in fact, the only way that a snake can fly is on a plane). The underside of these animals, which makes contact with the ground, is the key to their movement, because it determines how easy it is for them to slide.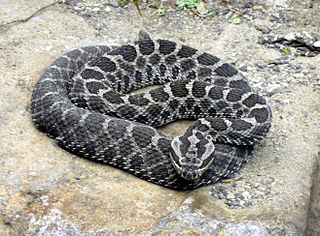
A close up view of a rattlesnake. Notice the scales covering the snake. A snake needs scales to help it slither.
Snakes have scales that aid them in moving forward, although it is difficult for a snake to move directly sideways. A snake gradually redistributes its body weight and uses its scales to provide thrust off of different points on the ground. There are four primary ways that snakes move: concertina, serpentine or lateral undulation, sidewinding, and rectilinear. That's a lot of movements for an animal without limbs.
Snails lack the scales that snakes have, and instead they expel mucus on which they slither. Similar to snakes, they move by utilizing a wave of muscular movement, and their adhesive mucus provides the friction necessary to avoid backsliding. Scientists have been studying the mucus-driven movement of snails to better design endoscopes, which must be inserted into the mucus-lined respiratory or digestive tracts.
Bipedalism
Bipedalism is the type of movement done on two limbs. Certain dinosaurs, such as the Tyrannosaurus rex, are thought to have been bipeds; it is suspected that bipedalism arose because it allowed the head to be maintained higher off of the ground to offer a greater range of vision. In addition, bipedalism allowed for specialization of forelimbs into wings or hands for flying or carrying things like tools and double cheeseburgers.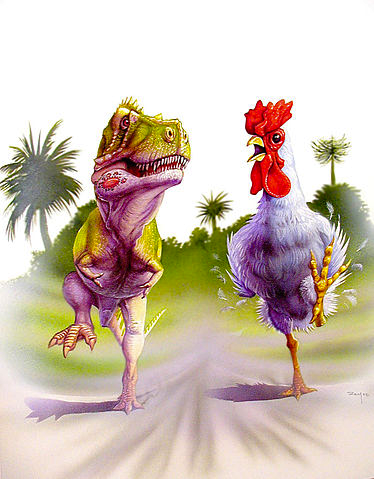
Cartoon illustrating the similarities between Tyrannosaurus rex and birds. Both are bipeds. Image from here.
Bipedal walking requires balance and the interplay of one foot pushing into the ground while the other foot propels forward. Some animals can also run, which is similar to walking, but in running there are moments when neither foot is touching the ground.
All birds are bipedal. For flightless birds like the ostrich and the emu this is their only way to move. Walking requires strong thigh musculature, and although birds may have a developed a negative reputation for having spindly "bird legs," they actually have large thigh muscles hidden close beneath their bodies. These strong muscles enable them to walk and hop around. Just check out the muscle on those turkey legs. If they weren't dinner, they'd probably be at the gym.
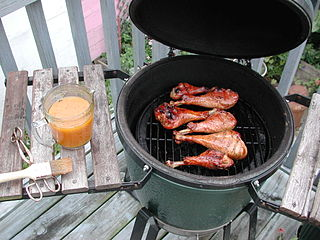
Turkey legs on a grill. Notice the thick upper thigh muscle. Image from here.
Humans walk differently than birds do because our bodies are not aligned the same. We stand fully upright with our spines vertically aligned and settled into our hipbones. Birds, on the other hand, have a spine that sits on their hips more like a T-shape with the neck and tail sticking out on either side of the central hip joint like the spine is planking on the hips. Remember planking? Weird.
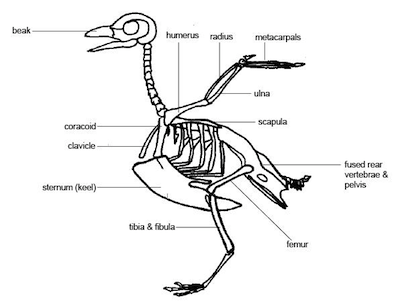
Diagram of a bird skeleton. Notice how the spine and rib cage are nearly perpendicular to the hips and legs. Humans are also bipeds, but they have a spine and rib cage that are in a straight line with their legs. Image from here.
Kangaroos are non-primate mammals that have adopted bipedalism, although they have not developed their forelimbs as much as birds and humans have. They also do not share the traditional walking gait of humans and birds and instead rely on hopping to get around at faster speeds. While grazing, a kangaroo's walking gait is neither bipedal nor quadrupedal. Its motion is pentapedal, where its tail acts as a fifth limb. It is able to form a tripod with its forelimb and its tail while its hindlimbs hop forward.
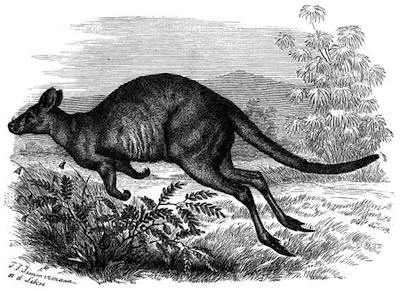
A kangaroo hopping on two large hind legs and using a tail for balance.
Quadrupedalism
Quadrupedalism is the movement of animals on four limbs. Because there are more feet, the movement becomes more complex. There are eight common quadrupedal gaits. They differ depending on when each foot hits the ground in relation to the other feet. Most animals, like horses and dogs, are capable of many gaits.1) Trot: Diagonally paired legs (such as front right and rear left) touch the ground simultaneously.
2) Bounding: Front (or back) legs touch the ground simultaneously.
3) Pace: Left (or right) side legs touch the ground simultaneously.
4) Walk: Each foot hits the ground in rotating order, front left, back right, front right, and back left, with three feet on the ground at all times.
5) Rotary gallop: Front feet hit at almost exactly the same time and then the back feet hit at almost exactly the same time. If the front feet hit right then left, then the back feet hit left then right.
6) Transverse gallop: Front feet hit at almost exactly the same time and then the back feet hit at almost exactly the same time. If the front feet hit right then left, then the back feet hit right then left.
7) ) Canter: Three beat type of movement. One foot hits, then the two remaining feet hit which are diagonal from each other, and then the final foot. For example—front right, then front left and back right, then back left. 8) ) Pronk: all four legs hit simultaneously. Yes, it's as cool as it sounds.
Since some animals use certain gaits but not others, animal trackers can use that information when they look at footprints. By looking at the shape, size, and the pattern of tracks, we can determine what kind of animal left them, how big it is, and how fast it was moving when it passed though. Hint: a flying animal will leave no tracks.
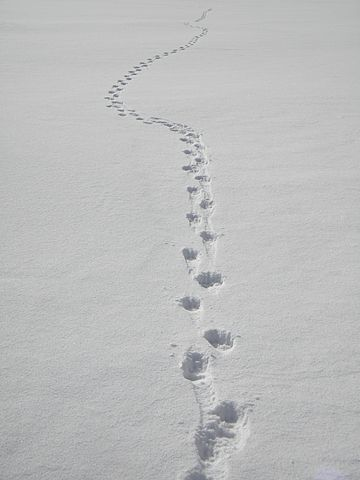
Tracks in the snow. Tracks can be examined to determine the gait that an animal had. Image from here.
Legs Legs Legs
Animals can have many more legs than four. Most insects have 6 legs, spiders have 8 legs, and centipedes and millipedes have hundreds of legs. Having more legs can be a hindrance, or a benefit.Scientists wondered what the benefit was for spiders to have eight legs instead of the traditional six legs. In the end, it took a dark room and a camera to figure it out. Amazingly, spiders are capable of walking flawlessly on six legs…in the dark. Almost like a blind person using a cane, they use their front pair of legs as antennae when they move in the dark.
There is a hindrance of having as many legs as a centipede or a millipede; it takes more coordination to move them all. Millipedes are relatively slow, but more legs means more power for pushing off the ground and for burrowing. Although the names centipede and millipede imply 100 legs and 1000 legs respectively, centipedes and millipedes don't actually have that many legs. Centipedes actually have between 30-362 legs and millipedes have between 36-400 legs, depending on the species.
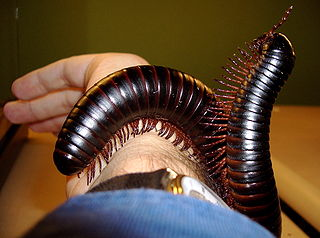
A Giant African Millipede. Look at all those legs. Image from here.
Why do Animals Move?
Predator/Prey
In the food chain, the object of the game is staying alive. Predators must move to catch prey, and prey must move to evade predators. Often, some animals have specific movements that they perform when hunting or avoiding predation. For example, killer whales have honed a skillful hunting maneuver to prey on sea lions in the surf zone. Although it is dangerous for a whale to beach itself, the sea lions are deliciously easy prey. Sea lions are skillful swimmers, but in the surf zone they need to hop along. There, they are at their most vulnerable. When a whale cruises onto the beach at 25 mph, they have little chance.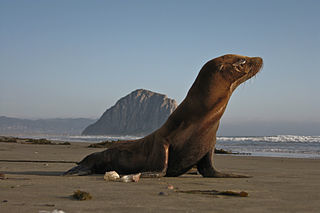
A sea lion pup on the beach. Sea lions can move on land or in the water, but they are built for water movement. On land they have to move very slowly. Image from here.
At the other end of the food chain, tiny fish have developed defensive movements. Median-paired fin movement allows fish to dart quickly in different directions. This helps them to avoid predators which cannot change direction as quickly.
Schooling or shoaling is another way that tiny fish can move to evade predators. Both tactics allow them to confuse predators with poor eyesight, and they can also provide safety in numbers since many eyes on the lookout makes it easier to identify potential predators.
Over time, predators and their prey can adapt counter-tactics to each other. One such tactic is demonstrated in an event known as the sardine run. Every summer, a large population of sardines can be found off the coast of Africa. Predators know that the sardines arrive every year, so they travel to the site, too. The sardines cluster together to protect themselves, but the predators have learned to benefit from this shoaling. They work together to round-up groups of sardines into tight clusters called baitballs. Then, the predators patrol the perimeter and take turns rushing in to satiate themselves at the swimming buffet. We bet there are some foods you wish would appear in large numbers every summer.
Social Movement
Animals also move for social reasons. Most animals are concerned with both self-survival and promoting the survival of their species. Finding a mate is very important, and there are many unique movements that animals display during courtship. Oh, love.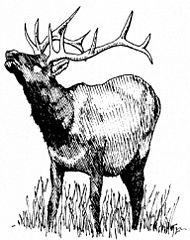
A male elk. Elk crash their antlers together in a physical competition for mating rights.
In many species, males compete with each other physically. For example, elk bound towards one another and crash their antlers together as they fight for mates. The bounding movement allows the elk to be more stable as he collides with his challenger, because it means he will dig in both front or back feet simultaneously, and ensuring greater balance.
Rather than fighting, many species of birds have complex mating rituals involving bizarre movements intended to draw attention of available females. Talk about lovebirds. Birds often fluff and displaying their vibrant plumage, although sometimes it can involve intricate dance-like movements as well. These guys have the moves like Jagger.
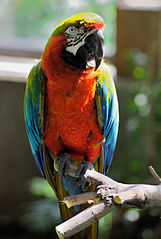
A colorful bird. Male birds are often brightly colored and perform complex movements to attract females. Image from here.
Migration
Another reason that people and animals move is to travel. This is particularly important for animals that live in changing climates. They enjoy their spring break as much as anyone. These animals sometimes need to migrate to specific locations at different times of the year.Many birds seek a warmer climate during the cold months, and, of them, bar-tailed godwits have the longest non-stop flight of any bird. By gliding on air currents and utilization of flapping flight, they have been known to fly from Alaska to New Zealand, a distance of 11,690 kilometers (or 7,263 miles) to take advantage of fair temperatures. In many parts of the United States and Canada, the crisp autumn air is filled with migratory Canada Geese in their classic V-formation. The flying V-formation is cost effective for birds traveling long distances because all birds can enjoy some of the lift caused by the lead bird. Flocks will rotate the front flier so that the burden of flying in the tiresome lead spot is shared. The flying-V is also one of the best offensive hockey maneuvers. Just ask the Mighty Ducks.
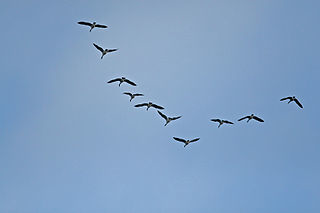
Canada Geese flying in a classic V-formation. Birds fly in this formation to conserve energy as a group. Image from here.
Caribou migrate similarly to birds except that they temporarily seek a cooler climate during the hot months rather than temporarily seeking a warmer climate during the cold months. They walk 965 km (600 miles) north during the summer months to feed on grasses of the tundra while avoiding the bloodsucking insects of warmer climates. Not a bad plan if you hate mosquitos.
Salmon have an even more complex migration pattern, as they travel to a special location for spawning. Utilizing strong body-caudal movement they propel themselves upstream against the current to spawn. Magically, they return to the exact stream where they were hatched. The distance and current alone make this a daunting task, but the truly amazing feat is when they propel themselves up a waterfall. Unfortunately, if there is a bear waiting at the top their effort will be wasted.
Brain Snack
1) Scientists are studying insect flight to create micro flying machines.2) While you're still thinking about flying sharks, could a shark moving super fast fly like an airplane? The short answer is, probably not. The shark's fins are angled in a way that generates lift when traveling through dense salt water, but the forces generated when moving through air would not be sufficient. Sharks can propel themselves up and out of the water momentarily, but fear not—flying sharks will remain in the Hollywood prop closet and out of the friendly skies. Or will they....